The Bohr Effect is a fundamental principle in physiology, shedding light on how our blood efficiently transports oxygen from the lungs to the body’s tissues while offloading carbon dioxide. Named after Danish physiologist Christian Bohr, who discovered it in the early 20th century, this phenomenon has critical implications for understanding respiratory physiology, especially during exercise and at high altitudes. In this comprehensive article, we’ll delve into the mechanics of the Bohr Effect, its historical context, molecular underpinnings, and significance in oxygen transport. We’ll also explore its applications in exercise physiology and adaptations at high altitudes.
Table of Contents
Christian Bohr and the Discovery of the Bohr Effect
To appreciate the Bohr Effect fully, we must begin with the pioneering work of Christian Bohr, a Danish physiologist who, in 1904, unveiled the intricate relationship between pH and the oxygen-carrying capacity of hemoglobin. Bohr’s groundbreaking experiments were instrumental in unraveling the mysteries of oxygen transport in the blood.
Christian Bohr was born into a family of accomplished scientists. His father, Niels Bohr Sr., was a renowned physiologist, and his brother, Niels Bohr Jr., would later become a Nobel laureate in physics for his contributions to atomic theory. Christian Bohr’s fascination with physiological research led him to investigate the properties of hemoglobin, the iron-rich protein found in red blood cells responsible for binding and transporting oxygen.
The Haemoglobin Molecule, a Molecular Transporter of Oxygen
To comprehend the Bohr Effect, one must first understand the hemoglobin molecule’s remarkable structure and function. Hemoglobin consists of four protein subunits, each containing an iron atom. When oxygen molecules bind to these iron atoms, hemoglobin becomes oxygenated, resulting in bright red blood. Deoxygenated hemoglobin, on the other hand, is darker in color.
The Bohr Effect Unveiled
At the heart of the Bohr Effect is the dynamic interaction between oxygen and hydrogen ions (protons). This intricate relationship can be summarized as follows:
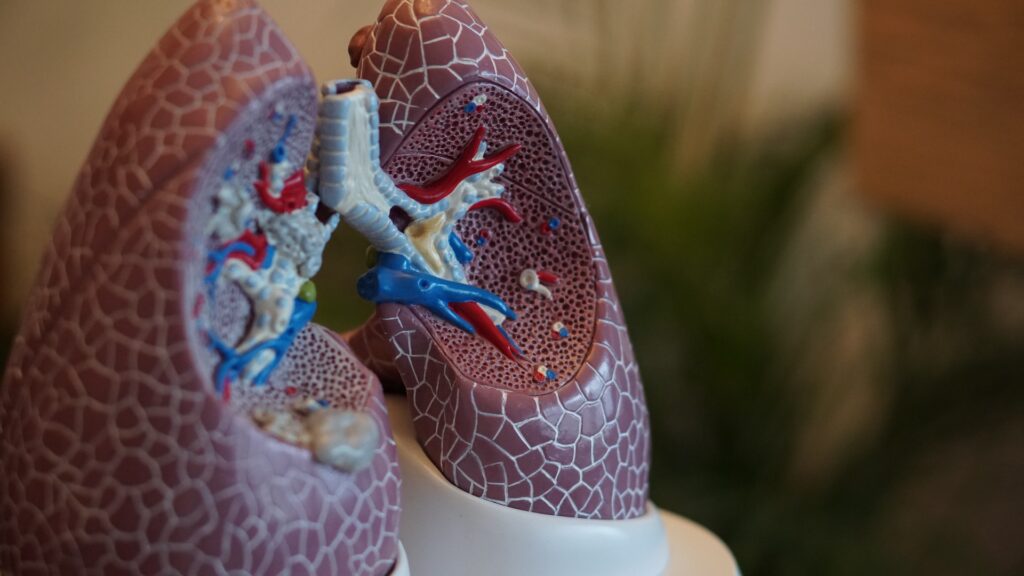
Lung Oxygenation
Blood arriving at the lungs is exposed to high oxygen levels, promoting the binding of oxygen molecules to hemoglobin. This forms oxyhemoglobin, which has a bright red color.
Tissue Oxygen Release
As blood flows away from the lungs and into the body’s tissues, it encounters varying levels of acidity and carbon dioxide concentration. These conditions favour the conversion of oxyhaemoglobin back into deoxyhaemoglobin, releasing oxygen where it’s needed most. The Bohr Effect ensures that oxygen is efficiently unloaded from haemoglobin in oxygen-deprived tissues.
The key factors at play in the Bohr Effect are pH and pCO2 (partial pressure of carbon dioxide). As pH decreases (indicating higher acidity), and pCO2 levels increase (indicating higher carbon dioxide concentration), hemoglobin’s affinity for oxygen decreases. In simpler terms, as blood becomes more acidic and carbon dioxide-rich, hemoglobin more readily releases its bound oxygen.
Significance in Oxygen Transport
The Bohr Effect plays a pivotal role in oxygen transport within the body. Without this phenomenon, our blood might struggle to efficiently deliver oxygen to oxygen-starved tissues. Let’s explore its significance:
Oxygen Delivery to Active Muscles
During strenuous exercise, muscles work harder and generate more carbon dioxide and lactic acid, leading to localized acidity. The Bohr Effect ensures that hemoglobin releases oxygen more readily in these oxygen-hungry muscle tissues, supporting peak physical performance.
Oxygen Release in Metabolically Active Tissues
In metabolically active tissues like the brain and heart, where cellular respiration generates carbon dioxide and lowers pH, the Bohr Effect helps oxygen to be released more readily from hemoglobin. This ensures that vital organs receive a steady supply of oxygen, even during increased metabolic demand.
Enhanced Oxygen Unloading in Hypoxic Conditions
At high altitudes, where oxygen levels are lower, the Bohr Effect helps compensate for reduced oxygen availability. Hemoglobin exhibits a greater tendency to release oxygen in response to the lower oxygen tension, ensuring sufficient oxygen delivery to vital organs. This adaptation is essential for individuals living or trekking at high altitudes.
Regulation of Blood pH
The Bohr Effect is not only crucial for oxygen transport but also contributes to the body’s pH regulation. By releasing protons (H+) during oxygen unloading, hemoglobin indirectly helps buffer excess acidity in metabolically active tissues.
Applications in Exercise Physiology
The Bohr Effect has profound implications for exercise physiology. Athletes and fitness enthusiasts can benefit from a deeper understanding of this phenomenon. Here’s how:
Enhanced Oxygen Delivery to Muscles
During exercise, muscles require more oxygen to sustain activity. Increased carbon dioxide production and acidity in active muscles trigger the Bohr Effect, promoting the release of oxygen from hemoglobin. This ensures that exercising muscles receive the oxygen they need to function optimally.
Delayed Onset of Muscle Fatigue
By facilitating oxygen delivery to muscles, the Bohr Effect contributes to delaying the onset of muscle fatigue during intense physical activity. This can help athletes achieve better endurance and performance.
Training at High Altitudes
Many athletes engage in altitude training to improve their performance. Understanding the Bohr Effect’s role in oxygen transport helps athletes adapt to lower oxygen levels at higher altitudes. This adaptation can lead to better performance upon returning to lower elevations.
High-Altitude Adaptations
In regions of high altitude, where oxygen levels are naturally lower, the Bohr Effect aids in oxygen delivery and tissue oxygenation. This adaptation is vital for populations living at high altitudes and adventurers who ascend to lofty peaks. Here’s how the Bohr Effect contributes to high-altitude adaptations:
Enhanced Oxygen Unloading
At high altitudes, where oxygen tension is reduced, the Bohr Effect ensures that hemoglobin readily releases oxygen to meet the body’s oxygen demands. Without this adaptation, individuals at high altitudes would experience severe oxygen deprivation.
Improved Oxygen Saturation
By facilitating oxygen unloading in the tissues, the Bohr Effect helps maintain adequate oxygen saturation levels in the blood. This is crucial for preventing altitude-related illnesses like hypoxia.
Acclimatization
Over time, individuals living at high altitudes undergo physiological adaptations, including adjustments in their blood’s oxygen-carrying capacity and the Bohr Effect. These adaptations help them thrive in oxygen-depleted environments.
Conclusion
The Bohr Effect, an elegant interplay between acidity, carbon dioxide, and haemoglobin, underpins our body’s ability to efficiently transport oxygen. Whether you’re an athlete striving for peak performance, a physiologist studying respiratory mechanisms, or an adventurer scaling high peaks, understanding the Bohr Effect provides insight into the remarkable precision of our physiology in oxygen transport. This intricate dance of protons and oxygen molecules within our blood ensures that the gift of life, in the form of oxygen, reaches every corner of our body, sustaining our existence and enabling our most extraordinary feats.
The counter part of the Bohr effect is Haldane Effect you can read more about it in our article name Haldane Effect A Key Player in Blood Gas Exchange. Also check the video how the Bohr effect work.
One comment
Comments are closed.