The Haldane Effect, named after the Scottish physician John Scott Haldane, is a vital physiological phenomenon that significantly impacts the transport of carbon dioxide (CO2) in the blood. While the Bohr Effect governs the binding and release of oxygen by haemoglobin, the Haldane Effect specifically relates to the influence of oxygen on CO2 transport. In this article, we will delve into the intricacies of the Haldane Effect, its historical context, molecular mechanisms, and its critical role in regulating blood gas exchange.
Table of Contents
The Pioneering Work of John Scott Haldane
The story of the Haldane Effect begins with John Scott Haldane, a remarkable scientist whose groundbreaking research in the late 19th and early 20th centuries revolutionized our understanding of respiratory physiology. Haldane’s experiments, often conducted on himself and his colleagues, laid the foundation for our knowledge of how the human body manages gases like oxygen and carbon dioxide.
The Role of Haemoglobin
To understand the Haldane Effect fully, it’s essential to revisit the role of haemoglobin. Haemoglobin, the iron-rich protein found in red blood cells, is responsible for binding and transporting oxygen (O2) and carbon dioxide (CO2) in the blood. Haemoglobin plays a dual role in this intricate dance of gases: it carries oxygen from the lungs to the body’s tissues and assists in the transport of CO2 from the tissues back to the lungs for elimination.
The Haldane Effect Unveiled
The Haldane Effect revolves around the oxygenation and deoxygenation of haemoglobin and its direct impact on the blood’s ability to carry and release CO2. It can be summarized as follows:
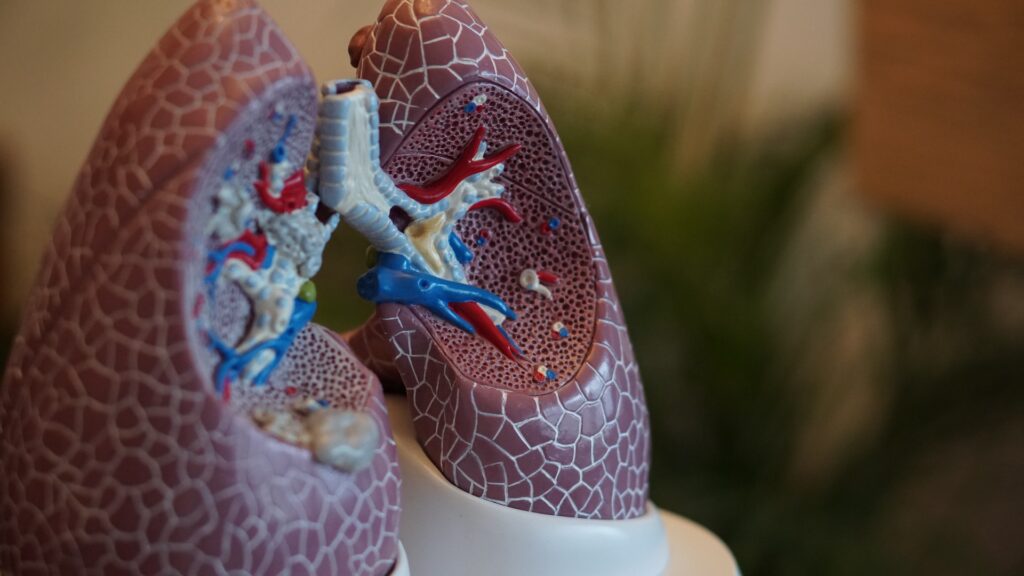
Oxygenation in the Lungs
When blood reaches the lungs, it encounters high oxygen levels. Haemoglobin in the blood binds with oxygen to form oxyhaemoglobin. This oxygenation process occurs in the pulmonary capillaries and leads to bright red oxygenated blood.
CO2 Unloading
The presence of oxygenated haemoglobin in the blood has a unique effect—it enhances the blood’s ability to release CO2. Haemoglobin’s affinity for CO2 is lower when it’s bound to oxygen. Therefore, in oxygen-rich areas like the lungs, haemoglobin releases CO2 more readily.
Deoxygenation in the Tissues
As oxygenated blood flows away from the lungs and into the body’s tissues, oxygen is unloaded from haemoglobin to supply the tissues. This deoxygenation transforms oxyhaemoglobin into deoxyhaemoglobin, which is darker in color.
CO2 Loading
Simultaneously, the deoxygenated haemoglobin becomes a more effective carrier of CO2. In tissues where CO2 is generated, deoxyhaemoglobin binds with CO2, forming carbaminohaemoglobin. This CO2 loading occurs most efficiently in areas where oxygen has been released.
Significance in Blood Gas Exchange
The Haldane Effect plays a critical role in maintaining the delicate balance of gases in the bloodstream. Here’s why it’s so significant:
Efficient CO2 Transport
The Haldane Effect ensures that CO2 generated during cellular metabolism is efficiently transported from the body’s tissues to the lungs for elimination. This helps prevent the build-up of excess CO2, which could lead to respiratory acidosis.
Facilitates Oxygen Unloading
By promoting the release of oxygen in oxygen-rich areas like the lungs, the Haldane Effect enhances the blood’s ability to supply oxygen to oxygen-starved tissues, such as actively exercising muscles.
Buffering Blood pH
The exchange of CO2 and oxygen mediated by the Haldane Effect indirectly influences blood pH. The release of CO2, along with the conversion of carbonic acid (H2CO3) to bicarbonate (HCO3-), contributes to the blood’s acid-base balance.
Clinical Relevance
Understanding the Haldane Effect is crucial in clinical contexts. It helps healthcare professionals interpret blood gas analyses, diagnose respiratory disorders, and make informed decisions about patient care. Disorders that affect oxygen and CO2 exchange, such as chronic obstructive pulmonary disease (COPD) and certain forms of pneumonia, can be better understood through the lens of the Haldane Effect.
Conclusion
The Haldane Effect, a companion to the Bohr Effect, is a cornerstone of respiratory physiology. Its elegant interplay with haemoglobin oxygenation and CO2 transport ensures that our bodies efficiently manage these vital gases. From its discovery by John Scott Haldane to its everyday clinical applications, the Haldane Effect continues to be a central concept in our understanding of how our bodies breathe and maintain gas balance, highlighting the marvel of human physiology.
One comment
Comments are closed.